Roundtable interview
Ambitious researchers explore the future of materials science
07/27/2015
With prestigious ERATO grants, two researchers at the AIMR plan to usher in a post-nanocarbon era, and create a new science of spin beyond spintronics
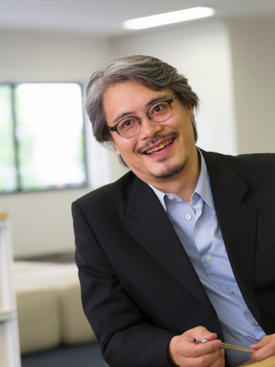
Organic chemist Hiroyuki Isobe and condensed matter physicist Eiji Saitoh at the AIMR have both received grants for projects under the prestigious research fund Exploratory Research for Advanced Technology (ERATO). Isobe’s laboratory, established in 2013, is creating new organic materials containing covalent bonds known as π bonds, which are commonly found in aromatic compounds such as benzene and DNA nucleotides. His team has begun incorporating these solid materials into organic devices with surprising results. Saitoh’s project was approved by the ERATO program in 2014. He aims to exploit the constant and unidirectional nature of spinning electrons to produce power sources for nanoscale mechanical devices.
AIMResearch spoke to both researchers about their ambitious goals and the importance of collaborative research.
AIMResearch: Professor Isobe, you have said that we are entering a ‘post-nanocarbon era’. Could you elaborate on what you mean by this?
Isobe: My undergraduate research involved isolating carbon-based structures known as fullerenes. We were very excited about these new spherical nanocarbon structures. Then, in the late 1990s, we were introduced to another type of nanocarbon called carbon nanotubes, also with unique properties. While fullerenes are molecular entities, the larger nanotubes are a mixture of various structures. One question remained in my mind — were these fullerenes and nanotubes really necessary to discover unique and extreme properties? Could such unique properties be developed using simpler aromatic systems? This is what I call the post-nanocarbon era, where chemists are inspired by nanocarbon structures to create discrete molecular versions of them through exploratory research.
AIMResearch: How is your Degenerate π-Integration Project under the ERATO program bringing this goal closer to reality?
Isobe: Our recent breakthrough came when we succeeded in synthesizing finite versions of carbon nanotubes using aromatic hydrocarbons. This led us to make a huge jump: we were able to explain the curved π-systems of nanocarbons and the origin of their uniqueness by using the well-developed language of chemistry. But this is just the beginning. We found that we could encapsulate spherical fullerenes in these carbon nanotubes with the highest association force ever observed in a supramolecular complex. Despite this strong force, the bearing is still free to rotate. This is a very exciting second step in our exploration of new phenomena or properties. We are currently looking for clues to take the third step.
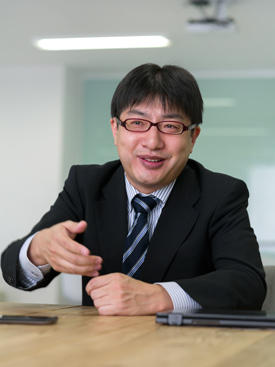
AIMResearch: Professor Saitoh, you have described your Spin Quantum Rectification Project under the ERATO program as ‘constructing a new paradigm of physics and matter’, in which you plan to expand concepts in quantum mechanics to the field of mechanics. Could you explain what you mean by this?
Saitoh: The field of spintronics emerged in the early 2000s as a new paradigm of electronics that uses electron spin in the same way that conventional electronic devices use electron charge, sometimes using both spin and charge in combination. Spintronics basically uses spinning electrons as small magnets, but it tends to overlook the fact that they actually rotate. I want to create the seeds for a new science of spin beyond spintronics.
Quantum mechanics says that an electron has spin and will rotate forever. We cannot stop this rotation. Thus, electrons can be thought of as very small motors — perpetually self-rotating motors — and can therefore be used to redirect fluctuations in matter to flow only in one direction, such as for the conversion of waste energy into useful energy. Take the famous Watt steam engine, for example. It consists of a water boiler and a rotating pump that redirects the random fluctuations of water molecules to produce mechanical motion. By embedding this natural rectification property of electron spin into nanoscale devices, we can provide a ubiquitous source of power for actuators or even create energy converters.
AIMResearch: What are the benefits of working at the AIMR and how do they add your work?
Saitoh: The degree of freedom. We have very few constraints here.
Isobe: The best thing about working at the AIMR is meeting brilliant guys like him! It’s really stimulating to hear them speak about concepts that I’m not familiar with.
Saitoh: I feel the same. The two of us occasionally exchange ideas and have even recognized some similarities in our research directions. His fullerene-and-carbon-nanotube bearing is a molecular rotator and mine — the spinning electron — is a quantum rotator. It might be a coincidence, but it smells interesting!
AIMResearch: We hear a lot these days about the importance of cross-disciplinary research. How significant is collaboration between researchers in different disciplines for your research?
Saitoh: My team consists of researchers from a wide range of backgrounds, including physicists, materials scientists, mathematicians and even cosmologists. Before joining the AIMR, I focused on physics — applying mathematics only to rationalize or formulate physical phenomena. But after joining the AIMR, I realized that as a way of thinking, mathematics has a lot to offer. Mathematicians want to know the structure or essence of a thing. Using mathematics, we have been able to discover similarities between two apparently different physical phenomena.
In quantum mechanics, there are only two objects for which the direction of time cannot be reversed — a black hole and a spinning electron. Just as electron spin can rotate in only one direction, a black hole can only expand, it cannot shrink. Mathematically, these concepts are very similar. Basically, we have to employ the same symmetry concepts as general relativity to fully understand why the angular momentum of an electron and the body hosting the electron remains constant ― this is a very new idea that no-one has thought about before. Mathematics allows us to discuss independent phenomena on an equal footing and gives us new insights into nature.
To implement these concepts, we need materials science and engineering. Our starting point has been the cutting-edge technology of very small machines known as microelectromechanical systems (MEMS). They lie at the intersection of theoretical physics and nanotechnology, but while MEMS are very popular among engineers, physicists don’t know much about them. We have already begun to exchange information to test whether our theoretically predicted phenomena can be deployed in practice using MEMS.
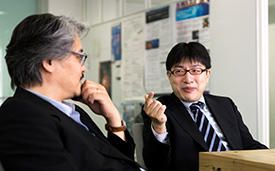
Isobe: Collaborative research is not about shouting at each other from opposite banks of a river ― it’s about stepping into the water to communicate more freely. This kind of collaboration has really benefited our group as well and has led to new molecular designs.
When researchers at Kodak first developed organic light-emitting diodes (OLEDs) in the early 1980s, they separated organic semiconductor materials into two types based on their understanding of conventional light-emitting diodes and inorganic materials — a ‘p-type’ hole transporter and an ‘n-type’ electron transporter. Commercial OLEDs are therefore made of five to six layers of different materials, each layer fulfilling a separate function to produce luminescence.
But it turns out that certain hydrocarbons can act as both electron and hole transporters. This discovery came out of an exchange between a materials scientist and an organic chemist in my group. The chemist asked: Do we really need an n- and p-type, since there is no such thing as ‘n’ and ‘p’ for organic molecules? The chemist was brave enough to question assumptions taken for granted in the other field. As a result, we have been able to develop an OLED device made of a single layer of one molecule.