Spintronics: A clean look at magnetic semiconductors
10/31/2016
Probing high-quality crystals with ultraviolet light reveals how spin-polarized electrons might power future low-energy memory devices
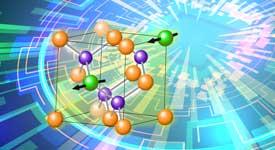
© 2016 Seigo Souma
An improved way to prepare ultraclean magnetic semiconductor surfaces that includes using a special ‘vacuum suitcase’ to move samples has helped AIMR researchers crack a two-decade-old puzzle regarding active charge carriers in these promising spintronic materials1.
Semiconductors made from gallium arsenide (GaAs) can exhibit intriguing properties when small amounts of manganese (Mn) atoms are incorporated into their structure. This is because the manganese dopants add permanent magnetic fields and positive-charge carriers to the semiconductor, allowing currents to be polarized into either up or down quantum spin states.
These characteristics have enabled researchers to use GaMnAs as a platform for new devices, such as spin transistors and magnetic memory, which can process information using minimal energy. However, understanding the carrier-induced magnetic properties of this semiconductor is tricky because effects from randomly distributed dopants are difficult to predict using standard quantum theory.
To settle this scientific debate, Seigo Souma of the AIMR at Tohoku University and his co-workers used a technique known as angle-resolved photoemission spectroscopy (ARPES) to directly probe the electronic band structure of GaMnAs and measure the ‘Fermi level’ at which dopant carriers are active.
In these ARPES measurements, high-intensity ultraviolet light stimulated crystals to emit electrons, which were analyzed to map out the relation between a charge carrier’s wave-like momentum and its energy. “This is the only way to determine the Fermi-level position of GaMnAs,” says Souma. “However, there are major problems inherent with its surface.”
Souma explains that since ARPES probes the upper few nanometers of a millimeter-sized scan area, it needs extremely clean and reliable surfaces. Unfortunately, merely exposing GaMnAs to air causes thick oxides to grow, which obscure the electron signal. The team overcame this problem by developing a suitcase capable of maintaining a vacuum nearly as devoid of atoms as outer space. They then used the suitcase to transport high-quality samples from a growth chamber to the ARPES system.
The ARPES results revealed that the Fermi level of GaMnAs lies deep within the electron-rich, or valence, band of the semiconductor — a location that helped the researchers select an appropriate theoretical model to describe the carrier-induced ferromagnetism.
Intriguingly, their measurements also showed that the intensity of emitted electrons depended on the orientation of an applied magnetic field, an effect known as magnetic linear dichroism. “Observing this effect with ARPES indicates strong spin−orbit coupling in our sample,” says Souma. “This is the main parameter needed to realize electric control over spin for computing and memory devices.”
References
- Souma, S., Chen, L., Oszwałdowski, R., Sato, T., Matsukura, F., Dietl, T., Ohno, H. & Takahashi, T. Fermi level position, Coulomb gap, and Dresselhaus splitting in (Ga,Mn)As. Scientific Reports 6, 27266 (2016). | article
This research highlight has been approved by the authors of the original article and all information and data contained within has been provided by said authors.