Metallic glasses: Local differences
05/30/2011
Nanoscale variations in the viscoelasticity of metallic glasses can help explain how they form and break
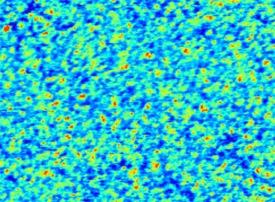
© 2011 APS
Most metals are crystalline in structure, with their constituent atoms arranged in a regular pattern. Amorphous or glass-like metals, on the other hand, have little or no atomic ordering, and thus have several advantages over crystalline metals. For example, they can be easily softened and shaped, and are often stronger and more deformable than a crystalline metal with the same composition.
Metallic glasses are formed by cooling a hot metallic melt fast enough to prevent its atoms from rearranging to form a crystal. They are highly conductive, like regular metals, but also exhibit enhanced strength and viscoelasticity. Although metallic glasses have been studied for decades, the initial idea that their properties are homogeneous has recently shifted towards heterogeneity at the nanoscale. Now, Mingwei Chen, Yanhui Liu and colleagues at the WPI-AIMR and the Institute for Materials Research at Tohoku University have characterized the nanoscale elastic properties of metallic glasses1.
The researchers used radiofrequency magnetron sputtering to form an atomically flat, 2 μm-thick film composed of the metals zirconium, copper, nickel and aluminum, and also showed the film to have a glassy structure.
The researchers then studied its surface using a technique previously developed by Ken Nakajima and Toshio Nishi, members of the research team, for polymeric materials, but they found that it was also suitable for metallic glasses. The technique involves moving a vibrating cantilever with a sharp tip, whose apex is just 1 nm in radius, across the surface of the metallic glass film. The tip interacts with the surface of the glass, thus allowing the viscoelasticity-induced energy dissipation of the material underneath the tip to be calculated from the phase change of the tip vibration. In addition, the topography of the glass surface is measured at the same time.
Chen and co-workers found that the viscoelasticity of their metallic glass film was not uniform, but instead varied by around 10% over distances of 3 nm (see image). In comparison, the same variation in film height occurred over distances of around 9 nm. The difference between these two numbers supports the interpretation that the phase data reflects inherent viscoelasticity, and is not related to surface roughness.
Heterogeneous viscoelasticity suggests that there are regions of the metallic glass that are more loosely packed than others, which may help scientists to understand how metallic glasses break and form. Regions of higher viscoelasticity, for example, are expected to deform more strongly under applied mechanical stress. “Our data bridges the gap between atomic modeling and macroscopic models of metallic glasses,” says Chen. “In particular, it may help us understand how metallic glasses undergo the transition to liquid-like phases.”
References
-
Liu, Y. H., Wang, D., Nakajima, K., Zhang, W., Hirata, A., Nishi, T., Inoue, A. & Chen, M. W. Characterization of nanoscale mechanical heterogeneity in a metallic glass by dynamic force microscopy. Physical Review Letters 106, 125504 (2011). | article
This research highlight has been approved by the authors of the original article and all information and data contained within has been provided by said authors.